Download The Urban And Regional Planning Reader Birch Pdf Converter
Abstract Our central paradigm for urban ecology is that cities are emergent phenomena of local-scale, dynamic interactions among socioeconomic and biophysical forces. These complex interactions give rise to a distinctive ecology and to distinctive ecological forcing functions. Separately, both the natural and the social sciences have adopted complex system theory to study emergent phenomena, but attempts to integrate the natural and social sciences to understand human-dominated systems remain reductionist—these disciplines generally study humans and ecological processes as separate phenomena. Here we argue that if the natural and social sciences remain within their separate domains, they cannot explain how human-dominated ecosystems emerge from interactions between humans and ecological processes. We propose an integrated framework to test formal hypotheses about how human-dominated ecosystems evolve from those interactions. For most of human history, the influence of human beings on biophysical processes, ecological systems, and evolutionary change has been relatively limited, as compared with the influence of “natural” (nonhuman) processes.
Ecological and evolutionary change has generally been attributable to natural variation in energy and material flows and to natural selection by parasites, diseases, predators, and competitors. Today, however, humans affect Earth's ecosystems at extraordinary rates through conversion of land and resource consumption (), alteration of habitats and species composition (), disruption of hydrological processes (), and modification of energy flow and nutrient cycles (, ). Humans now use approximately 40% of global net primary production () and more than half of accessible freshwater runoff ().
The Urban and Regional Planning Reader (Routledge Urban Reader Series) [Eugenie L. Birch] on Amazon.com. *FREE* shipping on qualifying offers. The Urban and Regional Planning Reader draws together the very best of classic and contemporary writings to illuminate the planning of cities and metropolitan areas. Search the world's information, including webpages, images, videos and more. Google has many special features to help you find exactly what you're looking for.
At least half of the world's forests have disappeared as a result of human activity, and three-quarters of that total have disappeared since 1700 (). Human activities fix amounts of nitrogen and sulfur comparable to those fixed by all nonhuman causes (). Humans have radically revamped Earth's carbon cycle () and freed into the environment vast quantities of naturally occurring trace materials (e.g., cadmium, zinc, mercury, nickel, arsenic) and exotic new anthropogenic substances (e.g., polychlorinated biphenyls, chlorofluorocarbons) (). Humans also influence evolutionary processes. Selection is more and more frequently directed by people, or at least by people interacting with other natural processes.
For example, humans affect speciation by challenging bacteria with antibiotics, poisoning insects, rearranging and exchanging genes, creating and dispersing thousands of synthetic compounds, and selectively fishing (). By hunting, moving predators and competitors around the globe, and massively reconfiguring the planet's surface, humans have increased extinctions of other species to levels 1000 to 10,000 times higher than those resulting from nonhuman causes (,, ). The combined effect of changing speciation and extinction is rapid evolutionary change (). Despite dominating Earth's ecosystems, humans remain conspicuously excluded as subjects of much ecological thinking and experimentation. Traditional ecological research investigates ecosystems in terms of biophysical, ecological, and evolutionary processes unaffected by human influences. During the last 100 years, formidable strides have been made in the scientific understanding of ecological systems ().
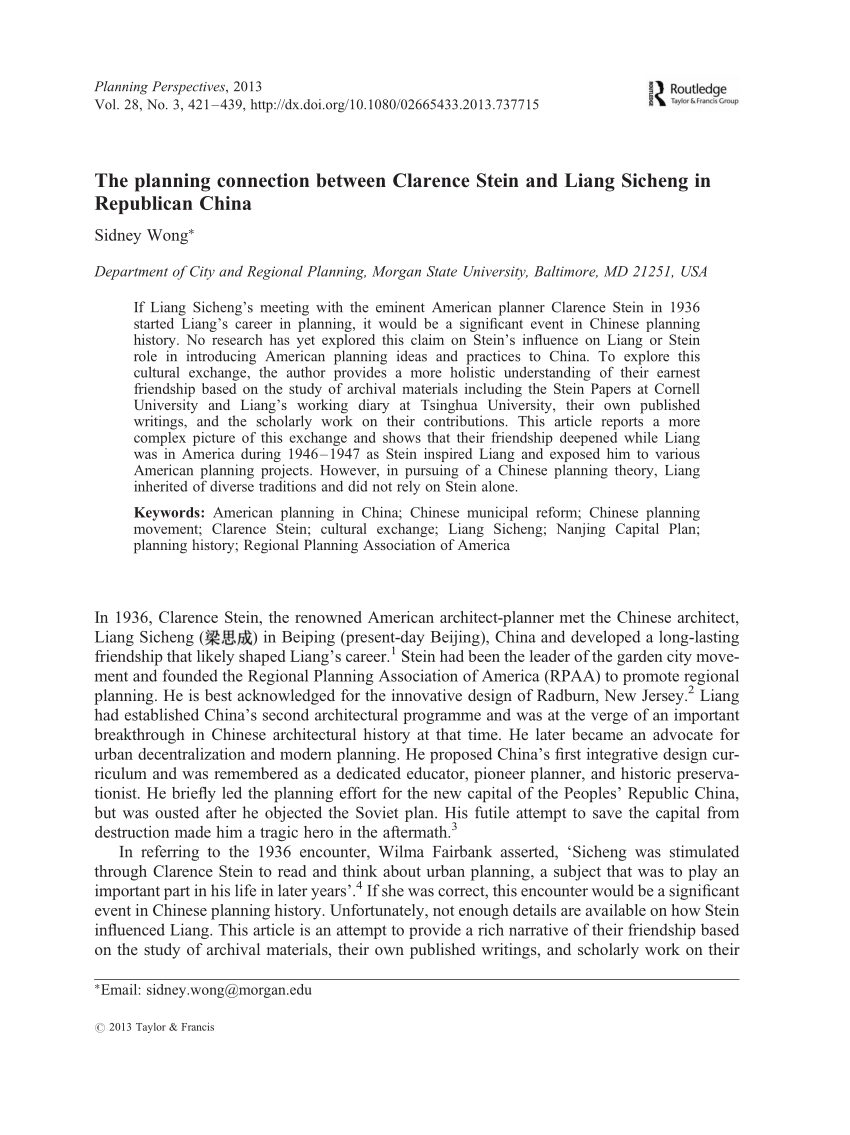
Evolutionary theory and population genetics have made fundamental changes in the assumptions underlying ecological research. Ecological scholars no longer regard ecosystems as closed, self-regulating entities that “mature” to reach equilibria. Instead, they see such systems as multiequilibria, open, dynamic, highly unpredictable, and subject to frequent disturbance (). In the newer non-equilibrium paradigm, succession has multiple causes, can follow multiple pathways, and is highly dependent on environmental and historical context. Ecosystems are driven by processes (rather than end points) and are often regulated by external forces (rather than internal mechanisms). The new ecological paradigm recognizes that humans are components of ecosystems (). Yet ecological scholars often fail to include humans in ecological science (,, ).
Applied ecology has extensively challenged the assumptions of an ecological paradigm that assumes human-free systems, but ecology has not yet provided a new theoretical framework to fully integrate humans into ecosystem studies. Here we argue that humans must be explicitly incorporated into all aspects of ecological thought, because, by adding powerful selection forces at every spatial scale and at many temporal scales, humans are fundamentally changing the expression of the rules that govern life on Earth. To paraphrase, humans are changing the ecological stage on which the evolutionary play is performed. To understand the new evolutionary play, ecological scholars must build a new stage with humans as a central plank.
Urban ecology: Understanding human-dominated ecosystems Planet-scale changes induced by humans are most evident in and around the urbanizing landscape (). Urbanized areas cover only approximately 1% to 6% of Earth's surface, yet they have extraordinarily large ecological “footprints” and complex, powerful, and often indirect effects on ecosystems. Earth's urban population has increased more than 10-fold over the past century, from 224 million in 1900 to 2.9 billion in 1999 ().
According to the United Nations (), all expected population growth from 2000 to 2030 (approximately 2 billion people) will be concentrated in urban areas. Arcgis Server Ecw Licensed here. By 2030, more than 60% (4.9 billion) of the estimated world population (8.1 billion) will live in cities. Ecological scholars studying urban areas have challenged ecological theory to explain the ecology in and of cities (). The urban long-term ecological research sites are now producing important empirical observations ().
Some have argued that important revisions to ecological theory are needed to include human activity (, ). To understand specific sets of interactions between humans and ecological processes that occur in urbanizing regions, we propose examining cities as emergent phenomena—phenomena that cannot be explained simply by studying the properties of their individual parts. Cities are both complex ecological entities, which have their own unique internal rules of behavior, growth, and evolution, and important global ecological forcing functions.
Cities as emergent phenomena. Ecology is a science of emergent phenomena: Populations have properties (birth and death rates) and behaviors (schooling in fishes, flocks of birds) not inherent in individuals. Like other ecosystems, cities are not the sum of their constituents; they are key examples of emergent phenomena, in which each component contributes to but does not control the form and behavior of the whole. Traffic congestion, air pollution, and urban sprawl emerge from local-scale interactions among variables such as topography, transportation infrastructure, individual mobility patterns, real estate markets, and social preferences. What makes urban regions different from many other ecosystems is that in these regions humans are a dominant component. Cities evolve as the outcome of myriad interactions between the individual choices and actions of many human agents (e.g., households, businesses, developers, and governments) and biophysical agents such as local geomorphology, climate, and natural disturbance regimes.
These choices produce different patterns of development (), land use (), and infrastructure density (). They affect ecosystem processes both directly (in and near the city) and remotely through land conversion, use of resources, and generation of emissions and waste. Those changes, in turn, affect human health and well-being (). We propose that resilience in cities—the degree to which cities tolerate alteration before reorganizing around a new set of structures and processes ()—depends on the cities' ability to simultaneously maintain ecosystem and human functions. Cities as complex ecological entities.
A diverse literature has begun to document some ecological characteristics of urban regions both in the United States (, ) and in Europe (, ). Human-dominated landscapes have unique biophysical characteristics. Humans redistribute organisms and the fluxes of energy and materials. The effects are both obvious (e.g., pavement) and subtle (e.g., conversion of forest to agriculture and then to suburbs; acid rain), both immediate (e.g., dams drown river valleys) and long-term (e.g., new intercity highways direct and promote city growth on 20- to 100-year scales). Relative to non–human-dominated systems, urban ecosystems have low stability, different dynamics (complex and highly variable on all temporal and spatial scales), more nonnative species, different species composition (often simplified, always changed), and unique energetics (antientropic in the extreme). They have rich spatial and temporal heterogeneity—a complex mosaic of biological and physical patches in a matrix of infrastructure, human organizations, and social institutions ().
Human activities directly affect land cover, which controls biotic diversity, primary productivity, soil quality, runoff, and pollution. Urbanized areas also modify microclimates and air quality by altering the nature of the land surface and generating heat (). Urbanization's increase in impervious surface area affects both geomorphological and hydrological processes; it changes fluxes of water, nutrients, and sediment (, ). Tmnt Dark Horizons Game Download here.
Because ecological processes are tightly interrelated with the landscape, the mosaic of elements resulting from urbanization has important implications for ecosystem dynamics. The transformation of land cover favors organisms that are more capable of rapid colonization, better adapted to the new conditions, and more tolerant of people than are many endemic, sensitive, locally specialized organisms. As a result, urbanizing areas often have novel combinations of organisms living in unique communities. Mixes of native and nonnative species interact in complex, anthropogenically driven successions, but with human participation, they also equilibrate into communities stable over time. Diversity may peak at intermediate levels of urbanization, at which many native and nonnative species thrive, but it typically declines as urbanization intensifies ().
Rearranging the pattern of land cover also changes the composition of communities; edge species, or those inhabiting interfaces among vegetation types and ecotones (such as white-tailed deer), typically increase, and interior species, or those rarely occurring within a few hundred meters of interfaces (such as northern spotted owls), decline (). Cities as global ecological forcing functions. The importance of cities as drivers of economic development has been recognized for a long time (), but their role as a global ecological driving force is not yet fully appreciated ().
Many ecological changes forced by cities on their immediate environments are obvious and extreme and have been extensively documented (). Although ecological impacts of urban development often seem to be local, urbanization also causes environmental changes at larger scales. Today's cities are sustained by a socioeconomic infrastructure that operates on global scales; the ecologically productive area required to support an urban area can be 100 to 300 times larger than the urban region (). Scholars have drawn on the concept of carrying capacity to propose ways to measure a city's ecological footprint (, ) and appropriated ecosystem area ().
Estimate the ecological footprint of Vancouver (British Columbia, Canada) at more than 200 times its geographic area; likewise, estimate that the appropriated ecosystem area required to supply renewable resources to 29 major cities in the Baltic Sea drainage basin is 200 times the total area of the cities. The spatial organization of a city and its infrastructure affect the resources needed to support the city's human activities and thus the city's level of environmental pressure on the regional and global environment (). The land development needed to house the same number of people varies, depending on choices about location, density, and infrastructure.
Whether an urban dweller chooses a private or public transportation system to commute between home and work, for example, depends on the availability of a public transportation system, which in turn depends on the political–economic feasibility of such a system, given the distribution of human activities. These choices have important ecological consequences globally and locally. Challenges for ecology The greatest challenge for ecology in the coming decades is to fully and productively integrate the complexity and global scale of human activity into ecological research. How can ecological scholars best study the complex biotic and abiotic interactions within human-dominated ecosystems, the emergent ecology of these systems, and their ecological forcing functions? We challenge the assumption that a “human-free” ecosystem paradigm can be productively applied to human-dominated ecosystems. We argue that leaving humans out of the ecological equation leads to inadequate explanations of ecosystem processes on an increasingly human-dominated Earth. Integrating humans into ecosystems will provide important opportunities for ecosystem science.
Consider, for example, how the key ecological concept of the niche could benefit from explicit inclusion of humans. Transformed and solidified the niche concept, changing it from a mere description of an organism's functional place in nature () to a mathematically rigorous n-dimensional hypervolume that could be treated analytically (). He also emphasized a single dimension of the hypervolume, interspecific competition. Hutchinson's “realized niche” included only those places where an organism's physiological tolerances were not exceeded (its “fundamental” niche) and where its occurrence was not preempted by competitors ().
Emphasizing competition in the niche concept distracted ecologists from investigating other potentially important community organizing forces, such as predation, resources variability, and human domination. A more complete understanding of ecological community assembly has begun to develop (), but it still lacks the inclusion of humans. We suggest that niche theory should distinguish realized from fundamental niches on the basis of human interaction (). Redefining the realized niche as an organism's hypervolume of occurrence in the presence of a gradient of human domination () would quantify the myriad ways humans force population-level ecological functions that structure communities.
Understanding the mechanisms of niche assembly in the presence of humans would allow ecologists to directly test the effects of competitors, predators, disease, and land-cover change on community organization, because these ecological processes are often manipulated by humans. The challenge for ecology is to define how humans differ in their effects on ecological processes and, through comparing these differences, to gain clearer insight into how nature works.
An integrated consideration of human interactions with food web complexity may shed light on another ecological contentious ecological principle: the influence of biological diversity on ecological stability. Human domination can increase food web complexity (e.g., by interspersing built and natural habitats; ), but this does not necessarily increase ecological or anthropogenic stability (i.e., resilience). Uncoupling the connection between diversity and stability in human-dominated ecosystems highlights the importance of species identity, rather than simply species richness, to community stability. Investigating the changing relationship between diversity and stability along a gradient of human domination can clarify when diversity begets stability, when diversity simply means unnecessary redundancy of ecological roles, and when diversity leads to instability (e.g., diversity resulting from importation of invasive exotics). Traditional ecological investigations of populations and communities could benefit from studying human-dominated ecosystems, as we suggest above. This has been shown, for example, by studies of the dynamics of nutrient cycling and energy flow that have begun to incorporate human domination (, ). These studies have enabled better prediction of ecosystem-level processes and have led to a greater appreciation of human influences on the planet.
A conceptual model for urban ecology Ecologists are paying increasing attention to the relationship between urbanization and ecosystems (,, ), but few have directly addressed how human and ecological patterns emerge from the interactions between socioeconomic and biophysical processes. Current study of urban ecosystems uses such simplified representations of human–ecological interactions that their system dynamics cannot be fully appreciated and understood. For example, most ecological studies treat urban areas as homogeneous phenomena and combine all anthropogenic factors into one aggregated variable (e.g., pollution load, population density, total paved area); thus, they represent urbanization as unidimensional.
This is unrealistic: Urbanization is multidimensional and highly variable across time and space. Socioeconomic studies, on the other hand, highly simplify and rarely discriminate among different and complex ecological and biophysical processes. This aggregate representation of human and ecological processes cannot explain human–environment interactions in human-dominated systems, nor can it allow ecological scholars to fully understand the complex dynamics of such systems, because many of these interactions occur at levels not represented in current integrated approaches (). Ecologists and social scientists have studied emergent ecological and social phenomena, but they have not explored the landscape-level implications of interactions between social and ecological agents.
In their separate domains, neither the natural nor the social sciences can explain how integrated human and ecological systems emerge and evolve, because human and ecological factors work simultaneously at various levels. Ecologists have studied self-organized patterns in social insect colonies composed of hundreds to millions of genetically similar individuals. These individuals interact locally, but collectively they produce large-scale colony dynamics that are not predictable from the individuals' characteristics. Urban planners, economists, and sociologists have described cities as self-organizing systems in which emergent bottom-up processes create distinct neighborhoods and unplanned demographic, socioeconomic, and physical clusters. The need to share local services and a customer base drives residents and businesses together, while competition for land, labor, and customers drives them apart.
Because of these forces, initial random distributions in human-dominated landscapes rearrange spontaneously into a self-organized pattern with multiple diverse clusters (). To fully integrate humans into ecosystem science, we propose a new conceptual model that links human and biophysical drivers, patterns, processes, and effects (). Although several new models address the relationship between urbanization and ecosystem dynamics (,, ), they do not explicitly represent the interactions between human and biophysical patterns and processes, nor do they represent the feedbacks from these interactions. In our model, both biophysical and human agents drive the urban socioeconomic and biophysical patterns and processes that control ecosystem functions. Using this framework, ecological scholars can ask questions about how patterns of human and ecological responses emerge from the interactions between human and biophysical processes and how these patterns affect ecological resilience in urban ecosystems.
This model can help test formal hypotheses about how human and ecological processes interact over time and space. It can also help establish (a) what forces drive patterns of urban development, (b) what the emerging patterns are for natural and developed land, (c) how these patterns influence ecosystem function and human behavior, and (d) how ecosystem and human processes operate as feedback mechanisms. Without a fully integrated framework, scholars can neither test hypotheses about the systems' dynamics nor produce reliable predictions of ecosystem change under different human and ecological disturbance scenarios.
Such knowledge is critical if managers and policymakers are to control and minimize the effects of human activities on ecosystems. An example: Urban sprawl Urban sprawl illustrates the complexity of interactions and feedback mechanisms between human decisions and ecological processes in urban ecosystems. Sprawl manifests as a rapid development of scattered (fragmented), low-density, built-up areas (“leapfrogging”; ). Between 1950 and 1990, US metropolitan areas grew from 538,720 square kilometers (km 2) (84 million people) to 1,515,150 km 2 (193 million people). Land development due to urbanization has grown 50% faster than population ().
Sprawl is driven by demographics (e.g., increases in numbers of households), socioeconomic trends (e.g., housing preferences, industrial restructuring), and biophysical factors (e.g., geomorphological patterns and processes) and is reinforced by infrastructure investment choices (e.g., development of highway systems; ). Sprawl is strongly encouraged by land and real estate markets () and is now a highly preferred urban living arrangement (). The phenomenon of sprawl shows how considering only aggregated interactions between humans and ecological processes cannot help explain some important mechanisms that drive human-dominated ecosystems.
Human decisions are the primary driving force behind environmental conditions in urban ecosystems, but these conditions cannot be explained by taking separately the behavior of individual agents (e.g., households, businesses, developers) competing in each market (e.g., job market, land and real estate market). Households, which are themselves complex entities, simultaneously compete in the job and real estate markets when deciding where to live. Furthermore, these agents have preferences and make tradeoffs that are highly dependent on biophysical factors.
Decisions about land development and infrastructure are strongly influenced by biophysical constraints (e.g., topography) and environmental amenities (e.g., “natural” habitats). From local interactions among these agents eventually emerge metropolitan patterns, which in turn affect both human and biophysical processes.
Resulting changes in environmental conditions then strongly influence some important human decisions. Furthermore, in these systems, uncertainty is important, since any departure from past trends can affect system evolution. Sprawl has important economic, social, and environmental costs (). It fragments forests, removes native vegetation, degrades water quality, lowers fish populations, and demands high mobility and an intensive transportation infrastructure.
Such environmental changes may eventually make suburban sprawl areas less desirable for people and may trigger more development at increasingly remote locations. But urban feedback is changed in form and is phase-lagged, often by decades (e.g., results of decisions on highway development). Municipalities are largely responsible for promoting sprawl. For example, cities often subsidize sprawl by providing public services (schools, waste disposal, utilities) that are priced independent of their real cost and distance from central facilities (), so that residents in the sprawled periphery usually do not pay the full costs of their own services ().
The “complex system” paradigm provides a powerful approach for studying urban sprawl as an emergent phenomenon and for devising effective policies to control its effects. Complex structures can evolve from multiple agents operating according to simple decision rules (, ). Some fundamental attributes of complex human and ecological adaptive systems—multiple interacting agents, emergent structures, decentralized control, and adapting behavior—can help scholars to understand how urbanizing landscapes work and to study urban sprawl as an integrated human–ecological phenomenon. Complex metropolitan systems cannot be managed by a single set of top-down governmental policies (); instead, they require the coordinated action of multiple independent players operating under locally diverse biophysical conditions and constraints, constantly adjusting their behavior to maintain an optimal balance between human and ecological functions. A research agenda for urban ecology We believe that a radical change is needed in how scholars frame questions about urban ecology. Urban ecological studies need to explicitly represent the complex urban landscape patterns if they are to answer questions about strategies for achieving more sustainable urban forms. Urban development is characterized by different land-use types (industrial, commercial, mixed use, single-family residential [SFR], multifamily residential [MFR], and open space), which exhibit different land-cover composition and configuration.
The Urban Ecology Team at the University of Washington is conducting a study that aims to shed some light on the impact of urban patterns on bird diversity and aquatic macroinvertebrates. Data source: IKONOS 2000. Urban ecological studies need to explicitly represent the complex urban landscape patterns if they are to answer questions about strategies for achieving more sustainable urban forms. Urban development is characterized by different land-use types (industrial, commercial, mixed use, single-family residential [SFR], multifamily residential [MFR], and open space), which exhibit different land-cover composition and configuration. The Urban Ecology Team at the University of Washington is conducting a study that aims to shed some light on the impact of urban patterns on bird diversity and aquatic macroinvertebrates.
Data source: IKONOS 2000. The fundamental niche of a species (those areas on Earth where its physiological tolerance limits are not exceeded) is an n-dimensional hypervolume, where each environmental gradient relevant to a species is one dimension (). (a) A two-dimensional view of two axes from the n-dimensional Hutchinsonian niche hypervolume for species A, which can exist in areas with moderate temperature and moderate relative humidity. We contend that theoretical ecologists have steered empiricists away from fully understanding how communities are assembled by emphasizing competition in the formalization of the niche concept. A better paradigm for understanding how biotic communities are structured is to document the effect of humans on species' realized niches.
(b) The Hutchinsonian realized niche is that portion of the fundamental niche not preempted by competitors, shown here for three species in the absence of people. (c) In this model of a realized niche with human involvement, Species A (e.g., a human commensal, such as the Norway rat) expands to fill its fundamental niche in the presence of people. Species B has a restricted distribution because the human-subsidized species A outcompetes it. Species C is intolerant of humans and is confined to portions of its fundamental niche where people do not exist.
Species D is imported by humans into the geographic niche space indicated in (c). As humans dominate more of Earth, the types of processes that assembled and structured this community (niche space) are fast becoming the rule rather than exception. The fundamental niche of a species (those areas on Earth where its physiological tolerance limits are not exceeded) is an n-dimensional hypervolume, where each environmental gradient relevant to a species is one dimension (). (a) A two-dimensional view of two axes from the n-dimensional Hutchinsonian niche hypervolume for species A, which can exist in areas with moderate temperature and moderate relative humidity. We contend that theoretical ecologists have steered empiricists away from fully understanding how communities are assembled by emphasizing competition in the formalization of the niche concept. A better paradigm for understanding how biotic communities are structured is to document the effect of humans on species' realized niches. (b) The Hutchinsonian realized niche is that portion of the fundamental niche not preempted by competitors, shown here for three species in the absence of people.
(c) In this model of a realized niche with human involvement, Species A (e.g., a human commensal, such as the Norway rat) expands to fill its fundamental niche in the presence of people. Species B has a restricted distribution because the human-subsidized species A outcompetes it. Species C is intolerant of humans and is confined to portions of its fundamental niche where people do not exist. Species D is imported by humans into the geographic niche space indicated in (c). As humans dominate more of Earth, the types of processes that assembled and structured this community (niche space) are fast becoming the rule rather than exception. An integrated model of humans and ecological processes to understand forces driving patterns of urban development, quantify resulting patterns of natural and developed land, determine how these patterns influence biophysical and human processes, and assess the resulting environmental changes and feedback on human and biophysical drivers.
In this conceptual model, drivers are human and biophysical forces that produce change in human and biophysical patterns and processes. Patterns are spatial and temporal distributions of human or biophysical variables.
Processes are the mechanisms by which human and biophysical variables interact and affect ecological conditions. Effects are the changes in human and ecological conditions that result from such interactions. In the diagram we provide some explicit examples of drivers, patterns, processes, and effects. For example, population growth in an area (driver) leads to increased pavement and buildings (patterns), leading to increased runoff and erosion (processes), causing lower water quality and decreased fish habitat (effects), which may lead to a new policy to regulate land use (driver). However, the same variable can fit into different boxes depending on the focus (issue, scale, and time frame).
For example, erosion is a process, but it can also be seen as a pattern that influences other processes such as nutrient cycles or as an effect resulting from runoff. An integrated model of humans and ecological processes to understand forces driving patterns of urban development, quantify resulting patterns of natural and developed land, determine how these patterns influence biophysical and human processes, and assess the resulting environmental changes and feedback on human and biophysical drivers. In this conceptual model, drivers are human and biophysical forces that produce change in human and biophysical patterns and processes. Patterns are spatial and temporal distributions of human or biophysical variables. Processes are the mechanisms by which human and biophysical variables interact and affect ecological conditions. Effects are the changes in human and ecological conditions that result from such interactions. In the diagram we provide some explicit examples of drivers, patterns, processes, and effects.
For example, population growth in an area (driver) leads to increased pavement and buildings (patterns), leading to increased runoff and erosion (processes), causing lower water quality and decreased fish habitat (effects), which may lead to a new policy to regulate land use (driver). However, the same variable can fit into different boxes depending on the focus (issue, scale, and time frame). For example, erosion is a process, but it can also be seen as a pattern that influences other processes such as nutrient cycles or as an effect resulting from runoff.